CRISPR: A New Tool to Help Understand Cancer-Linked Mutations
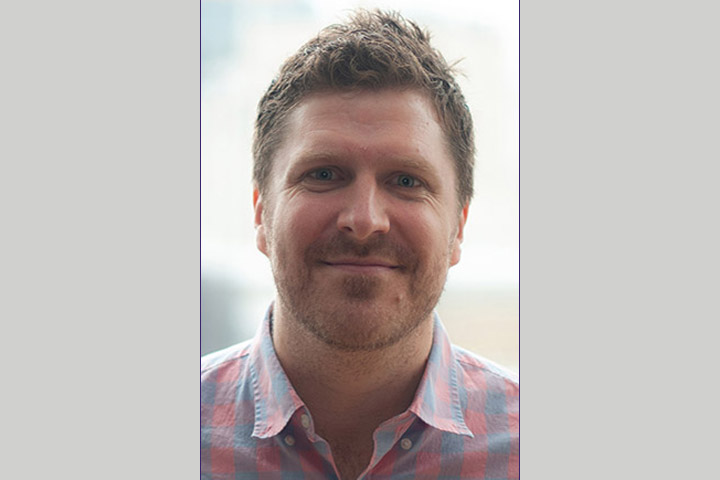
Slightly more than a decade ago, a new technology called CRISPR became the hottest new biology breakthrough since the discovery of DNA back in the late 1860s.
CRISPR is an acronym that stands for “clustered regularly interspaced short palindromic repeats.” This complicated term simply means that there are repeated sections in bacteria’s DNA. These repeats are actually copies of tiny pieces of viruses. Bacteria want to get rid of those viruses, so they put an enzyme called Cas9 to work. Cas9 cuts through the DNA like a pair of scissors, effectively chopping up the DNA in specific locations.
That elegant process, which is essentially a bacterial immune system response, gave scientists a bold idea: to use CRISPR to edit the genome of living organisms by adding, removing, or rearranging fragments of DNA. This technology offers scientists a programmable way to replace a disease mutation with a healthy sequence, knock out a gene entirely to study its function, or even modify portions of the genome that affect expression and gene function. The ultimate goal is to treat disease and answer fundamental questions in biology.
CRISPR and Cancer
But when it comes to fighting cancer, CRISPR has some limitations. Although CRISPR is “fast and simple,” the basic tools are “not that precise,” explains Lukas Dow, Ph.D., an associate professor of biochemistry in medicine at Weill Cornell Medicine in New York City. “Cancer is a very complex disease. Even though we can use Cas9 to cause insertions and deletions that can ablate the function of a gene, we couldn’t mimic the variants—the single nucleotide variants—that are most common in human cancers.” That means a change in just one letter in the code that makes up a cancer-linked mutant-variant gene can significantly affect how aggressive a tumor is or how well a patient with cancer may respond to a particular therapy.
That led Dow and his team at Weill Cornell to develop a new, more precise gene-editing tool that will allow scientists to study the impact of these specific genetic changes in preclinical models rather than being limited to broader targets, such as deleting the entire gene.
“Cas9 is very good and very efficient at cutting DNA,” says Dow, who is also a member of the Sandra and Edward Meyer Cancer Center at Weill Cornell Medicine. “You get alleles that aren’t random, but they aren’t easy to control. Imagine a book where every word represents a gene in our genome. Cas9 is great at removing words, but more often than not cancer mutations just change individual letters. Removing a word or changing a letter can have a huge impact on how a sentence is read. To understand the ‘book of cancer’ we have to be very precise with these subtle changes so that we can better predict how a cell responds to treatment.”
About the Study
The tool was described in a recent edition of the journal Nature Biotechnology. In the research, Dow and his colleagues genetically engineered mice to carry an enzyme—an engineered derivative of Cas9—that allows the scientists to change a single base, or “letter,” in the mouse’s genetic code. This “base editing” enzyme can be turned on or off by feeding the mice an antibiotic called doxycycline, reducing the prospect of unintended genetic changes occurring over time. Investigators can also grow organoids—miniature versions of intestine, lung, and pancreas tissue—from the mice, enabling even more molecular and biochemical studies of the impact of these precise genetic changes.
“If you want to understand a specific patient’s response to therapy, you first have to understand the genetic changes that influence that response,” explains Dow. “That’s one of the tenets of personalized medicine.”
Differences in a single base in a gene can have functional consequences. But most gene-editing tools currently available aim at larger targets like whole genes. Scientists can also use viruses to deliver genes with specific mutations, but this technique is limited to targeting specific tissues like the brain and liver, Dow adds. The mouse model Dow and his team developed allows them to study the effects of the changes on tumors and determine which therapies work best for those with a particular mutation. Organoids derived from the mice enable detailed experiments in tissues they could not easily target with virus-based approaches.
“One mouse model allows you to do two things: test the effects of a mutation in cancer initiation, progression, or treatment response in mice, and take a closer look at the associated molecular or biochemical changes using organoids,” Dow says.
The team is currently using this new technology to identify the effects of single-base mutations in pancreatic cancer as well as lung and colon cancers.
Sharing the Tech
The team’s genetically engineered mice will be available to other researchers across the world to use in their own studies. “This is the right thing to do,” explains Dow. “Science must be a collaborative endeavor. It would be complete hubris to think we can make positive changes in the lives of cancer patients without the expertise and research of other scientists. We can do far more together than we could ever do alone.”
He is also excited about how the technology may be used in the future. “I’m excited, but there’s a long way to go. It’s a lot of hard work, and we’re still scratching the surface. But we’re making steady progress,” he says.
“We’re climbing a mountain. Hopefully these tools make the journey a little faster.”